What caused the transition in style during the subglacial rhyolitic eruption of Dalakvísl
Dalakvísl is a volcanic edifice in Torfajökull, South Iceland (see map). It formed 70,000 years ago, with several other edifices, during Iceland's largest known eruption of subglacial rhyolite, under an ice sheet approximately 300 m thick. There are both explosively and effusively generated deposits, however, field evidence and geochemistry suggests that Dalakvísl formed within a single event. This suggests that during the formation of Dalakvísl there was a transition in style. But was it a transition from explosive to effusive behaviour of vice versa? And why did this change in behaviour occur?

Photographs from Dalakvísl showing (a) an overview, (b) a lava lobe which formed effusively (non-violently), (c) an obsidian sheet which is thought to have formed during the transition in style and (d) ash formed through explosive fragmentation (Owen et al., 2013).
In Owen et al., (2013a) it was discovered that the more explosively formed deposits had higher pre-eruptive water concentrations and likely experienced CSD: closed system degassing (where the gas remains within the magma) and fast magma ascent rates. By comparison, the effusive samples had much lower pre-eruptive water concentration, and show evidence of OSD: open system degassing (where the gas is lost from the magma en route to the surface) and slow magma ascent rates. (Click here to read more about this).
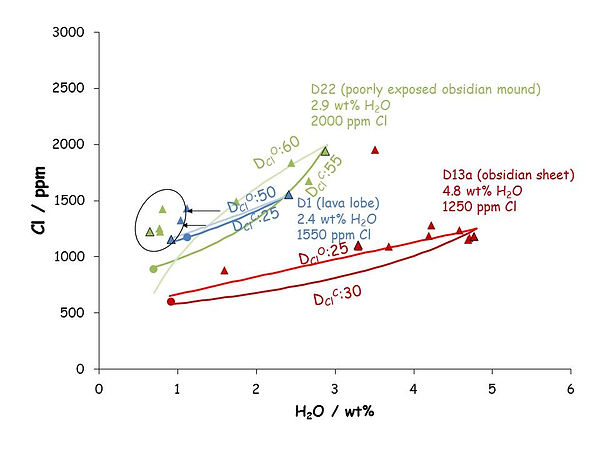
Initial H2O and Cl concentrations measured with SIMS. The lines show modelled degassing paths for OSD (paler colours) and CSD (darker colours) using the initial volatile contents and Cl distribution ratios as labelled. The circled measurements show those suspected of showing diffusive loss (Owen et al., 2013b)
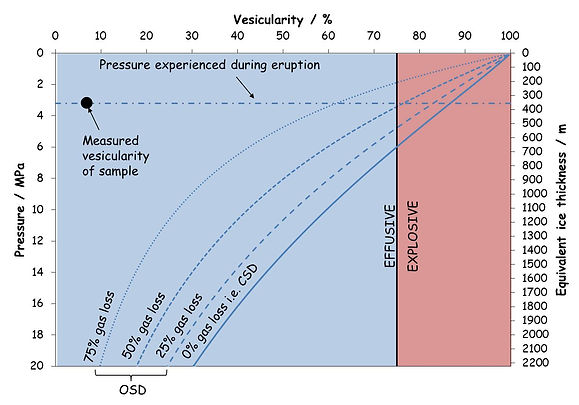
Modelled fragmentation depths for one of our effusive samples. The expected bubble content (vesicularity), based on its initial water content, is plotted against pressure (i.e. depth) for closed system degassing (CSD) and various degrees of open system degassing (OSD). When the bubble content reaches 75% the magma should fragment. The dash-dot line shows the pressure the magma erupted at (based on the dissolved water content of the sample) and the black circle shows the measured vesicularity of the sample. Our model predicts that in this case, the magma should have fragmented if it had lost up to 50% of the gas en route. If it had lost 75% of the gas it should have had a vesicularity of ~62%. The fact that we measured 7% suggests that the vast majority of the gas escaped en route. If this sample had experienced CSD it would have erupted explosively (modified from Owen et al., 2013b).
We also modelled fragmentation depths. In theory, magma will fragment when the bubble content reaches 75%. Bubbles grow as magma rises up the conduit. The final bubble content upon eruption will depend on (a) the initial gas content (b) the pressure experienced by the magma when it erupts and (c) the amount of gas that is lost en route.
We can measure the initial gas content using melt inclusions (click here for more information) and we can infer the pressure felt by the rock as it cooled using the dissolved water concentration (click here for more information). However, we don't know how much gas is lost en route so we modelled 0, 25, 50 and 75%.
We found that for the effusive samples, the measured vesicularity was significantly lower than would be expected for CSD. Furthermore if these samples had erupted with CSD, they should have reached the critical bubble content (vesicularity) and therefore fragmented, by the time the magma rose to the pressure at which the eruption took place.
This is further evidence that the effusive parts of Dalakvísl experienced open system degassing.
We tried to reconstruct how thick the ice was over Dalakvísl when it erupted. To do this we plotted various solubility pressure curves (which represent hypothetical ice thicknesses) against the matrix glass water content which we measured using FTIR.
However, we discovered that the Dalakvísl data plotted to two solubility pressure curves (SPCs). The effusive samples plot to a SPC representing high pressure, whilst the more explosive samples plot to a SPC which represents lower pressure.
The zone 1, sheet samples (red squares) plot in-between the two SPCs. The way that they don't fit to a SPC suggests that the degassing of these samples could not keep up with the pressure change (i.e. degassing was in disequilibrium), therefore the pressure change must have been very rapid.
The most likely explanation is that a jökulhlaup (glacial flood) caused a rapid reduction in pressure. If the meltwater could not drain away freely, meltwater could have accumulated near the vent. A 130 m drop in water level, would explain the pressure difference suggested by the measured water contents.
Seeing as effusive samples formed under the higher pressure regime, this would mean that the transition at Dalakvísl was from effusive to explosive behaviour. Reductions in pressure have been known to trigger more explosive behaviour: e.g. the 1996 Gjálp eruption saw an increase in activity following a pressure drop caused by a jökulhlaup.
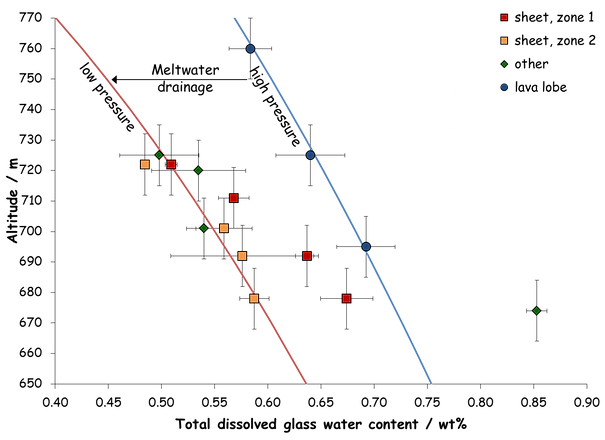
Dissolved glass water content (measured with FTIR) plotted against elevation. The curved lines are solubility pressure curves (SPCs) which show the water content expected at each elevation for various inferred pressure regimes. Lava lobes (blue circles) are thought to have formed effusively and all fit to a SPC representing high pressure. The water content of the obsidian sheets (squares) suggests that they formed under lower pressure. (Modified from Owen et al., 2013b)
Conclusions
Effusive samples
Low initial H2O
Open system degassing
Slow magma ascent
High loading pressure
Explosive samples
High initial H2O
Closed system degassing
Fast magma ascent
Low loading pressure
Also...
* The transition at Dalakvísl was from effusive to explosive behaviour
* If the effusive samples hadn't experienced open system degassing, then they should have fragmented in an explosive manner (so degassing path was obviously an important controlling factor but was change in degassing the trigger for the transition?)
* The pressure change was likely the result of rapid meltwater drainage in a jökulhlaup (this has been known to trigger a transition in style during other eruptions but did it do so at Dalakvísl?)
...
It is unresolved what triggered the transition in style at Dalakvísl , however, one model that explains all the data is as follows.
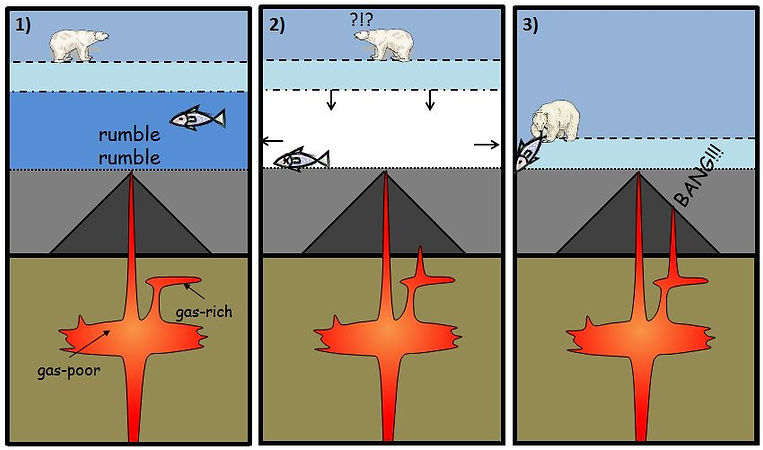
1) The eruption of Dalakvísl began under thick ice/water. The magma chamber has a low H2O content so the magma rises slowly, allowing gas to be lost en route (open system degassing). All of this means that the eruption behaviour is effusive (non-violent)
2) Meltwater that had accumulated near the vent-site suddenly drains away in a jökulhlaup. This rapidly reduces the pressure, opening up fracture networks which changes the magma source
3) The eruption is now under thin ice and fuelled by much more gas-rich magma. The gas helps the magma to rise quickly, which prevents gas-loss en route (closed system degassing). Consequently the eruptive behaviour is now explosive
If you have any comments or questions, please post them on the bottom of the page or contact me
The literature behind it:
This webpage is a summary of the findings of Owen, J., Tuffen, H. and McGarvie, D.W. (2013b) Pre-eruptive volatile content, degassing paths and depressurisation explaining the transition in style at the subglacial rhyolitic eruption of Dalakvísl, South Iceland, Journal of Volcanology and Geothermal Research, 258, 143-162
Other recommended reads:
Owen, J. (2013) Volatiles in Icelandic subglacial rhyolite, PhD thesis, Lancaster University
Owen, J., Tuffen, H. and McGarvie, D.W. (2013a) Explosive subglacial rhyolitic eruptions in Iceland are fuelled by high magmatic H2O and closed-system degassing, Geology, 41(2), 251-254
Tuffen, H., McGarvie, D. W., Pinkerton, H., Gilbert, J. S., and Brooker, R. A. (2008) An explosive-intrusive subglacial rhyolite eruption at Dalakvísl, Torfajökull, Iceland, Bulletin of Volcanology, 70(7), 841-860
Tuffen, H., Owen, J. and Denton, J. (2010) Magma degassing during subglacial eruptions and its use to reconstruct palaeo-ice thicknesses, Earth-Science Reviews, 99(1-2)
Stevenson, J.A., Gilbert, J.S., McGarvie, D.W. and Smellie, J.L. (2011) Explosive rhyolite tuya formation: classic examples from Kerlingarfjöll, Iceland, Quaternary Science Reviews, 30(1-2), 192-209
McGarvie, D.W. (2009) Rhyolitic volcano-ice interactions in Iceland, Journal of Volcanology and Geothermal Research, 185(4), 367-389
Humphreys, M.C.S., Edmonds, M., Christopher, T. and Hards, V. (2009) Chlorine variations in the magma of Soufrière Hills Volcano, Montserrat: Insights from Cl in hornblende and melt inclusions, Geochimica et Cosmochimica Acta, 73(19), 5693-5708.
Jaupart, C. (1998) Gas loss from magmas through conduit walls during eruption, in The physics of explosive volcanic eruptions, edited by J. S. Gilbert and R. S. J. Sparks, Geological Society Special Publication, No. 145, 73-90.
Jaupart, C. and Allègre, C.J. (1991) Gas content, eruption rate and instabilities of eruption regime in silicic volcanoes, Earth and Planetary Science Letters, 102(3-4), 413-429.