What controls the explosivity of subglacial rhyolite?
A subglacial volcano is any vent that is covered by snow or ice. It could be a snow-capped stratovolcano like Snæfellsjökull or a volcano covered by a glacier like Katla.
Ice and magma is an explosive combination so subglacial eruptions have a reputation for being quite disruptive. The 2010 eruption of Eyjafjallajökull, demonstrated this well when fine grained ash, generated by the interaction between magma and ice, was blasted into the atmosphere grounding nearly all European and some Trans-Atlantic flights.

Katla

Snæfellsjökull
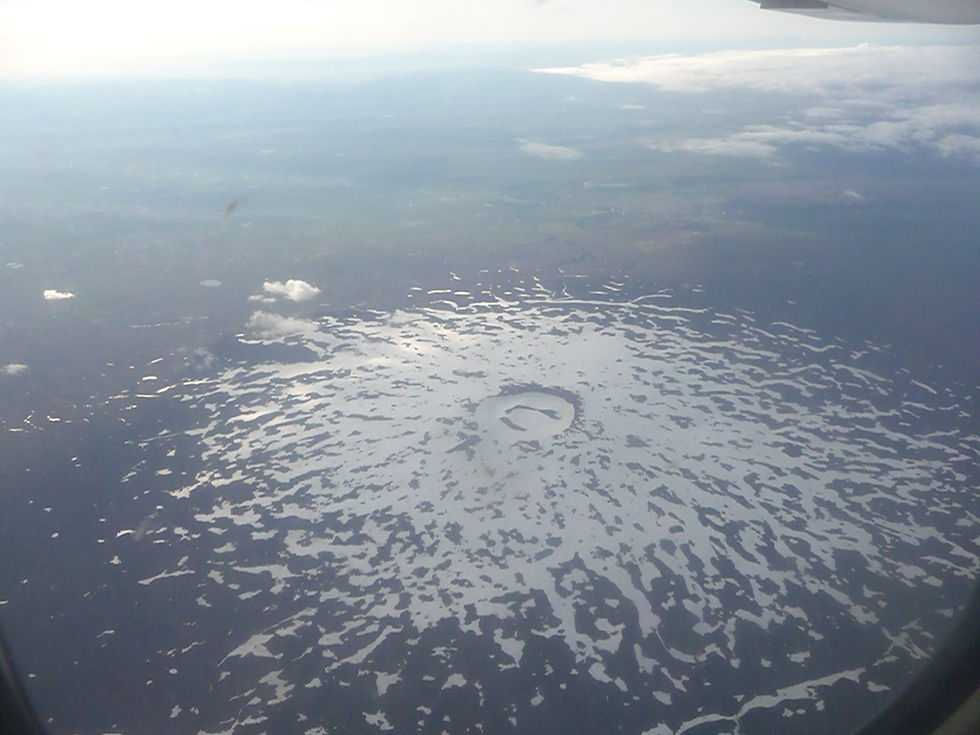
Ok

Katla
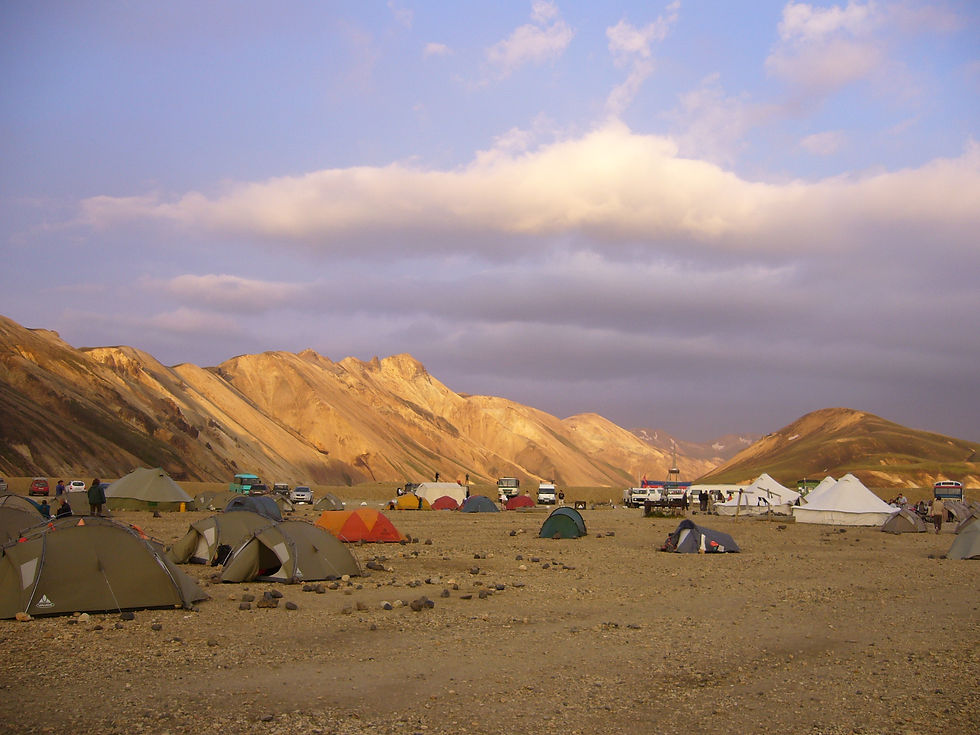
Landmannalaugar
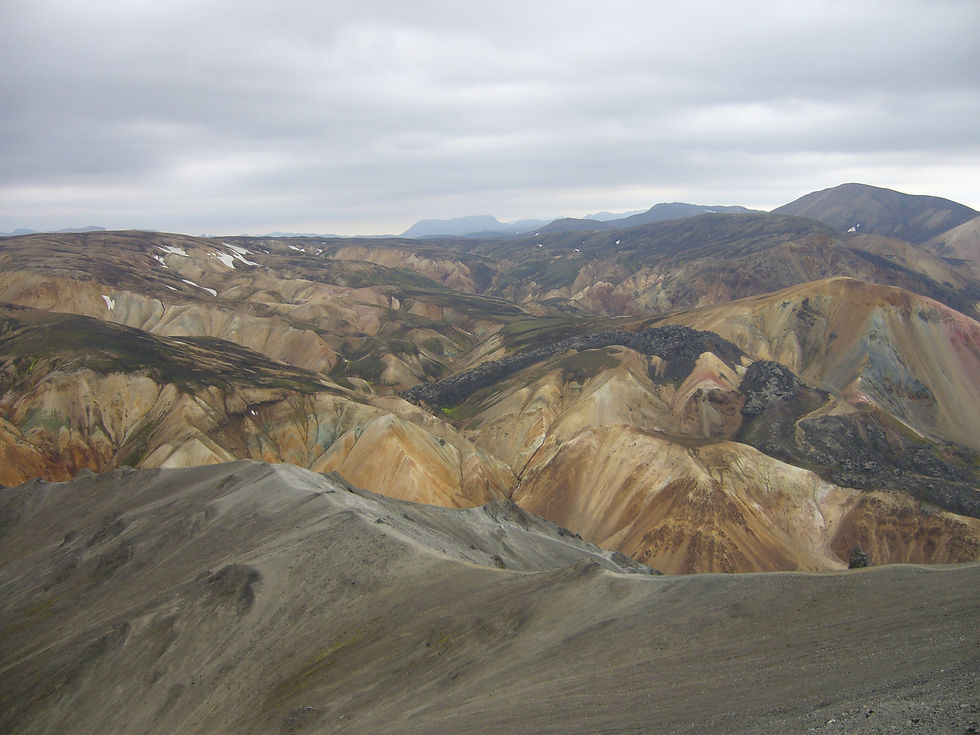
Landmannalaugar
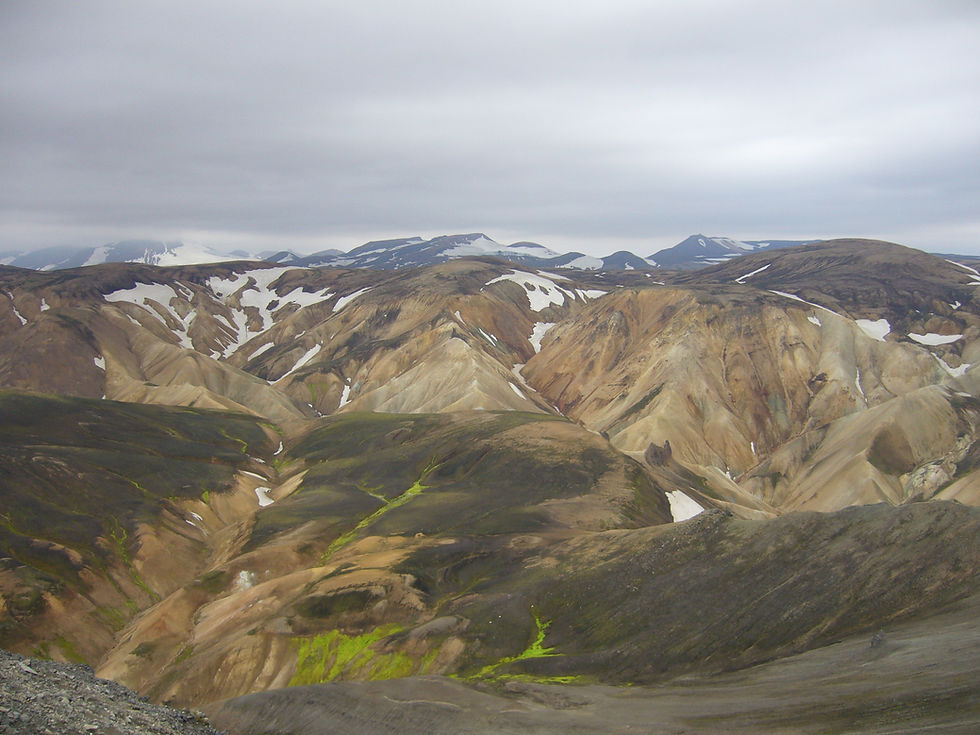
Landmannalaugar
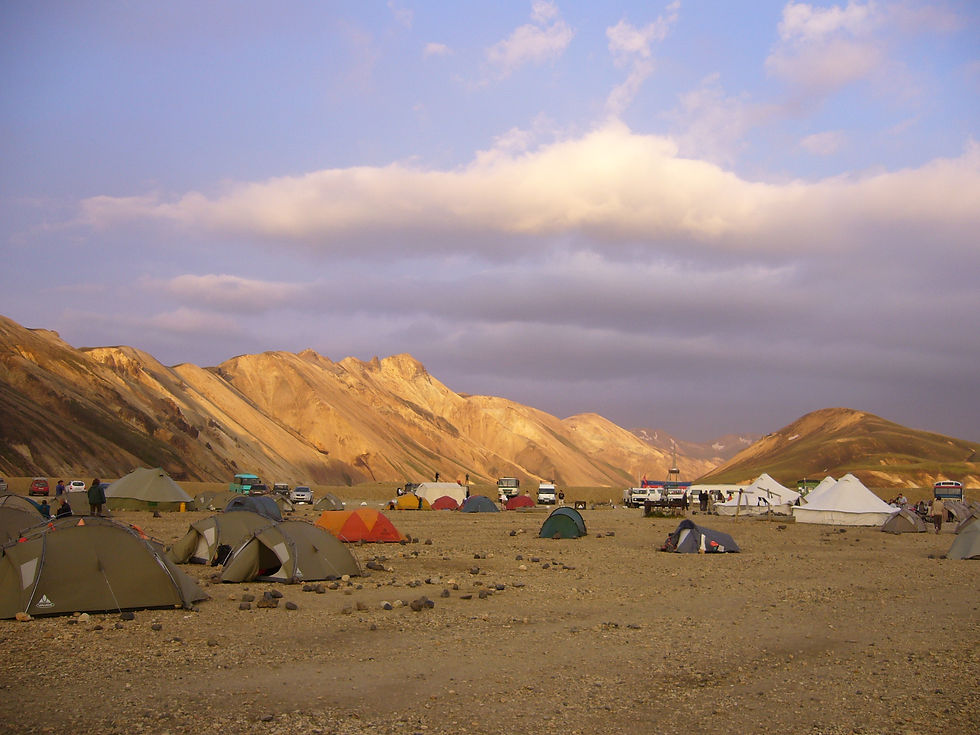
Landmannalaugar
Rhyolite is a type of magma which contains a lot of silica. It is the other end of the spectrum from basalt which contains comparatively little silica. Silica reduces the ability of the magma to move. Therefore, rhyolite doesn't always make it to the surface but often solidifies underground as granite. When rhyolite does erupt, it tends to do so quite explosively. It's resistance to flow means that a lot of pressure can be stored up which then tears the magma apart when pressure is finally released. By comparison, basalt tends to readily flow away from the vent in lava flows and fire fountains (unless it erupts under ice).
Rhyolite is much rarer than basalt but in Iceland makes up 10% of the rocks and at Kerlingarfjöll and Torfajökull it is 25% and 80% respectively, producing beautiful orange mountain scenery (see left).
So subglacial + rhyolite = explosive?
Well not necessarily! Surprisingly, subglacial rhyolitic edifices show a whole spectrum of eruptive styles. A subglacial rhyolitic eruption has never been observed but field evidence suggests that some subglacial rhyolitic edifices were formed by predominantly explosive activity whilst others suggest effusive (non-violent) activity. Some edifices even show evidence for a change in style during the eruption? So what is controlling the explosivity of subglacial rhyolite?
To find out we compared 5 edifices at Torfajökull, south Iceland (see map). These edifices were formed with contrasting eruptive styles but are all rhyolite and all subglacial. In fact all of the edifices with the exception of Bláhnúkur were thought to have erupted as part of the same eruption: the Ring Fracture eruption which happened 70,000 years ago and is Iceland's largest known subglacial rhyolite eruption. So why the different behaviour?
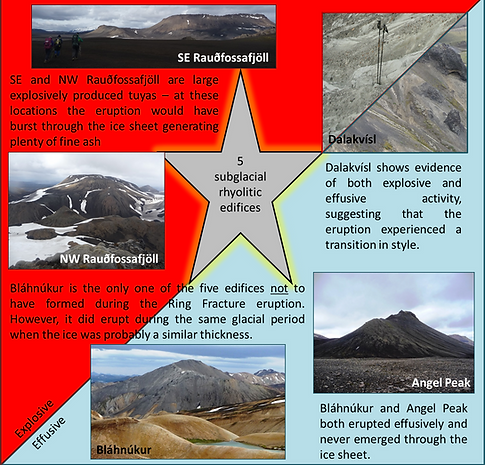
We used secondary ion mass spectrometry (SIMS) to measure the initial water content preserved in melt inclusions.
The explosively formed edifices had significantly higher initial water contents compared to the effusive edifices. This suggests a strong link between explosivity and initial water content.
Furthermore, water contents close to 5 wt.% were measured. This is much higher than was thought possible for Icelandic rhyolite which is often described in the literature as being 'dry'.
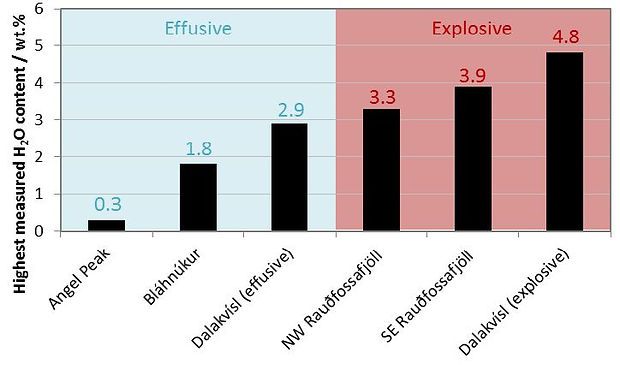
(Owen, 2013)
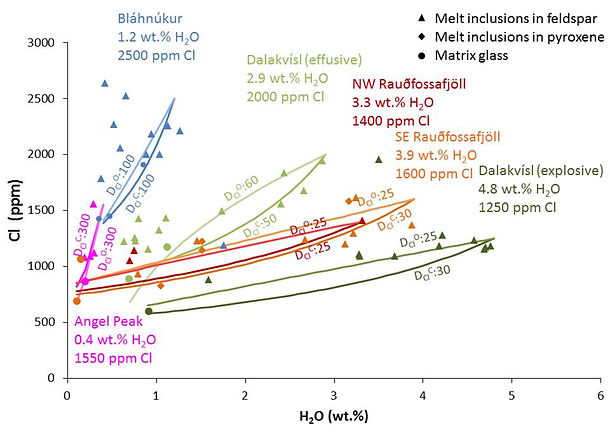
When magma rises up to the surface, it will either experience closed system degassing (where the gas remains within the magma) or open system degassing (where the gas is lost from the magma en route).
We tried to model closed system degassing (CSD) and open system degassing (OSD) as shown on the left. However, for each edifice, it was not clear from the data which modelled degassing path (CSD or OSD) provided the best fit to the data.
However, it was noticeable that the effusive edifices (i.e. Bláhnúkur, Angel Peak and the effusive parts of Dalakvísl) required much higher chlorine distribution coefficients (Dcls) than the explosive edifices (i.e. NW Rauðfossafjöll, SE Rauðfossafjöll and the explosive parts of Dalakvísl).
Initial H2O and Cl concentrations measured with SIMS. The lines show modelled degassing paths for OSD (paler colours) and CSD (darker colours) using the initial volatile contents and Dcls as labelled (modified from Owen et al., 2013)
Chlorine distribution ratios (Dcls) represent the amount of Cl in the fluid phase compared to the amount dissolved in the melt. One of the causes of a higher Dcl is crystallisation. When we look at our samples in thin section we note that our effusive samples have considerably more microlites (tiny crystals) compared to our explosive samples.
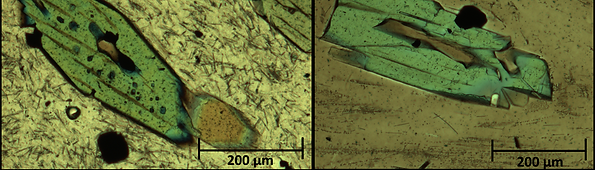
Typical thin sections from an effusive sample (left) which is full of microlites (thin black lines within the matrix glass) and from an explosive sample (right) where the matrix glass is almost microlite free (modified from Owen et al., 2013)
Microlite growth is encouraged by degassing (because volatile-loss changes the melting point of the magma) and favours slow ascent rates (because it allows time for the microlites to grow). Open system degassing both promotes gas loss and is linked to slower ascent rates. Therefore, there is a strong link between microlite-rich magma and magma that has experienced open system degassing.
We therefore propose that the effusive edifices experienced open system degassing with slow ascent rates, whereas the explosive edifices experienced closed system degassing and fast ascent rates.
Conclusions
Explosive volcanism is fuelled by high water contents and closed system degassing!
We found a strong link between volcanic gasses and explosivity. For the five subglacial rhyolitic edifices we studied at Torfajökull, the explosive edifices have high initial H2O contents and show evidence of closed system degassing. The effusive samples have low initial H2O contents and show evidence of open system degassing. We found no link between explosivity and the inferred ice thickness. This suggests that is is volcanic gasses, not ice, that is controlling the explosivity of these eruptions.
John Stevenson came to a similar conclusion when studying subglacial rhyolite at Kerlingarfjöll (click here to read his blog)
We also discovered that Icelandic rhyolite is much more water-rich than previously thought. These discoveries are worrying. It could potentially mean that anywhere where there is rhyolite in Iceland, there is potential for highly explosive volcanism, regardless of ice thickness.
If you have any comments or questions, please post them on the bottom of the page or contact me
The literature behind it:
This webpage is a summary of the findings of Owen, J., Tuffen, H. and McGarvie, D.W. (2013) Explosive subglacial rhyolitic eruptions in Iceland are fuelled by high magmatic H2O and closed-system degassing, Geology, 41(2), 251-254
Other recommended reads:
Owen, J. (2013) Volatiles in Icelandic subglacial rhyolite, PhD thesis, Lancaster University
Stevenson, J.A., Gilbert, J.S., McGarvie, D.W. and Smellie, J.L. (2011) Explosive rhyolite tuya formation: classic examples from Kerlingarfjöll, Iceland, Quaternary Science Reviews, 30(1-2), 192-209
McGarvie, D.W. (2009) Rhyolitic volcano-ice interactions in Iceland, Journal of Volcanology and Geothermal Research, 185(4), 367-389
Humphreys, M.C.S., Edmonds, M., Christopher, T. and Hards, V. (2009) Chlorine variations in the magma of Soufrière Hills Volcano, Montserrat: Insights from Cl in hornblende and melt inclusions, Geochimica et Cosmochimica Acta, 73(19), 5693-5708.
Jaupart, C. (1998) Gas loss from magmas through conduit walls during eruption, in The physics of explosive volcanic eruptions, edited by J. S. Gilbert and R. S. J. Sparks, Geological Society Special Publication, No. 145, 73-90.
Jaupart, C. and Allègre, C.J. (1991) Gas content, eruption rate and instabilities of eruption regime in silicic volcanoes, Earth and Planetary Science Letters, 102(3-4), 413-429.